Hidden Worlds: a peek into the nanoscale using helium ion microscopy
By Erin Grant
How do scientists know what happens at scales smaller than you can see using an optical microscope? One exciting method is the helium ion microscope which can be used to view cells, crystals and specially engineered materials with extreme detail, revealing the beauty that exists at scales too small to imagine!
Edited by Jessica Nguy and Hamish Payne
Issue 2: December 10, 2021
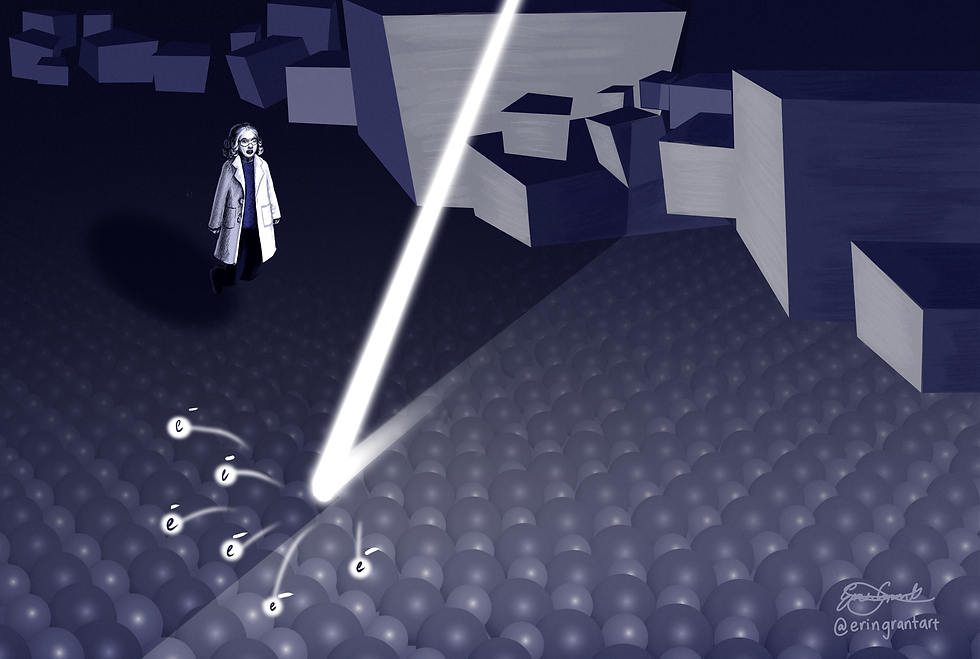
Illustrations by Erin Grant
The room is white, with three smooth walls and a fourth containing a small sample prep bench and high shelves. In the centre is a desk with three monitors. Next to it, occupying most of the space, is the microscope. Eight feet tall, a few feet wide, resting on an isolated floor surrounded by caution tape; “NO STEP” written in big block letters. Wires protrude from its tiered shape in orderly chaos. It is a clean, technological space; we are ready to explore science.
A colleague and I are at the Materials Characterisation and Fabrication Platform of the University of Melbourne to finish off the last steps of a scientific paper I’ve been working on for many years. What I need, as the icing on the cake, is an image. What does my sample look like way down there, at the nanometre scale?
Objects that are only nanometres in size are very hard to imagine when we’re used to thinking about metres, centimetres, or maybe even millimetres. We can see those length scales; they are part of our everyday. So, if you’re told that proteins have a diameter of a few nanometres, what does that mean?
Well, to be precise, a nanometre is one-billionth of a metre. A human hair, the go-to yardstick for describing small things, has a width between 0.05-0.1 millimetres, which means that if you wanted to slice a hair into nanometre-wide strands you’d end up with nearly 100,000 pieces. Unfortunately, that’s still hard to visualise, but I’ve found that when working with and thinking about scales like this every day, you gain a sort of mental landscape that small things occupy, perhaps not entirely in context, but a space that contains an overall ‘vibe’ of smallness.
I first noticed this when I worked in a laboratory that studies the tiny nematode worm C. elegans. These creatures are half a millimetre long, so although they are clearly visible to the naked eye, you need a microscope if you want to use them for science. After looking at these tiny creatures under magnification for many weeks, I came to recognise a feeling almost like being underwater. Upon putting my eyes to the lens, my focus would change from the macroscopic world around me, to one of minutiae. This change in perspective was quite immersive, I almost felt like I was inhabiting that small petri dish too.
Working with samples even smaller than that now, I have carried some of that mental landscape with me. It now feels commonplace to imagine tiny systems, such as crystals or molecules which were once foreign. Much of this ability to visualise small things comes from the fact that in many cases, we can actually see them too. Physics has given us many tools with which we can peer into the smallest systems that exist. Helium ion microscopy, which I have come here to carry out, is one such technique.
Dr Anders Barlow runs the helium ion microscope (HIM) at this facility. He warmly welcomes me and my colleague into the quiet room and jumps straight into an enthusiastic explanation of the machine – he can tell we’re not just here for some pictures, we want to know the inner workings of the microscope too.
The HIM is a bit like the more mature surveyor of minuscule worlds: the electron microscope. While a regular optical microscope uses light to illuminate a sample, the electron microscope uses electrons. When they collide with the sample these electrons can bounce off or lose energy through several mechanisms. The lost energy can go into heat or light, but more usefully, the energy might be transferred to other electrons in the sample, called secondary electrons, ejecting them like a drill removing rocks from a quarry.
The secondary electrons can be detected at each point across the sample as the beam is scanned over its surface. If more electrons are detected, then the pixel at that point is brighter compared to areas where there are fewer electrons. This tells you about the topography or composition of the sample at that point on its surface and provides a grayscale image.
The HIM works in the same way, but it can generate sharper images because helium ions are heavier than electrons. This is important because the increased resolution of electron and helium ion microscopes is enabled by their quantum mechanical properties - namely the particle’s wavelength. You may have heard about the wave-like nature of light, which is a basic property of quantum mechanics. Particles also have a wavelength, called the de Broglie wavelength, which is inversely proportional to their mass - the heavier the particle, the shorter the wavelength. Having a shorter wavelength allows smaller details to be resolved because of a pesky phenomenon called diffraction.
Diffraction occurs when a wave encounters a gap that is of the same or smaller width to its wavelength. When this happens, the wave that emerges on the other side will be spread out. You can think of the features that you want to image as being similar to gaps, so when light, or a particle, interacts with features that are very close together it will spread out, making those features blurry or even invisible. But if you can ensure that the wavelength is smaller than whatever feature you want to see, diffraction will not occur. Interestingly, physicists can actually take advantage of diffraction, and another phenomenon called interference, when they study periodic structures like crystals, but that’s a different article!

So, because the de Broglie wavelength is very short for particles with mass, like electrons, an electron microscope can generate images of higher resolution than an optical microscope. Likewise, helium ions are even heavier than electrons because they are composed of one electron, two protons, and two neutrons. This makes them about 7,000 times heavier than a single electron (electrons are very light compared to protons and neutrons!) and consequently the images they can make are very sharp.
With our samples ready, lab manager Anders loads my sample into the microscope and begins lowering the pressure in its internal chamber. Having a high vacuum – approximately a billion times lower than atmospheric pressure – is essential because it prevents air from interfering with the helium beam.
Making the beam is perhaps the most miraculous part of this technological feat. At the very top of the microscope’s column, there’s a tiny filament shaped like a needle. Not like a needle, in fact, it is the sharpest needle we humans can make. To achieve this, the point is shaped by first extreme heat, and then some extreme voltages until the very tip is composed of only three atoms, reverently referred to as the trimer. Once the trimer has been formed, a high voltage is applied to the needle, resulting in an extreme electric field around the tip. Next, helium gas is introduced into the chamber and individual helium atoms are attracted towards the region of the high electric field. The field is so strong that it strips each helium atom of one electron, ionising it, and these now positively charged ions are repelled from each of the three atoms in the trimer as three corresponding beams.
Using sophisticated focusing fields down the length of the column allows Anders to choose only one of the beams for imaging; we are creating a picture using a beam only one atom wide!
Generating such a precise beam requires constant maintenance, but once Anders is satisfied with how it looks today, he begins scanning over a large area for what we’ve come to find: tiny proteins stuck to a diamond.
In an experimental PhD, you often find yourself answering small incremental questions and today I want to know how well I’ve attached these proteins to my diamond and what the coverage looks like. Other measures have told me that I probably have a lot of them, but the best way to know is to have a look! That’s what Anders does for researchers at the university; he helps us find out whether we have done a good job putting things together or coming up with new techniques. This is something he loves about his job.
“I love the exposure I get to many areas of science,” he says, “Imaging of all forms is ubiquitous in research, and the HIM is applicable to most fields, so we see samples from materials science, polymers, nanomaterials, and biomaterials, through to medical technologies and devices, to cell and tissue biology of human, plant and animal origin. I never get tired of seeing what new specimens may come through the lab door.”
Unfortunately, the first images we see are very dark and washed out, like a photograph taken in low-light; not many secondary electrons are making it to the detector. To combat this, Anders uses a flood gun to stop charge build up on the surface of the diamond. When the helium ions create secondary electrons, they are ejected from the surface at low speeds. As electrons are negatively charged, the bombarded surface, which now lacks electrons, will become positive and the low energy secondary electrons will be attracted back to the surface instead of making it to the detector.
In an electron microscope this is avoided by coating insulators, such as my diamond, with a conductive material like gold. If the surface is conductive, the positive charge that is left behind by the secondary electrons will be offset by electrons from the metallic coating that can flow towards the sudden appearance of positive charges. In this case, the ejected electrons can escape and be detected. However, a coating like this would reduce the resolution of the image; if you want to measure proteins that are twelve nanometres high, but you put a three-nanometre coating over them, you’ll lose a lot of the resolution! To get around this, the HIM uses the flood gun, which lightly sprays the surface with electrons of low energy as the helium beam passes over. This neutralises the surface and lets the secondary electrons escape in the same way as having a conductive layer.

Once Anders turns on the flood gun, the contrast increases, allowing us to zoom in on a small region of the diamond, and there they are! Thousands of spherical proteins arranged neatly across the surface, only twelve nanometres in diameter. The sight is spectacular, only one try and we got what we came for.
I am three years into a PhD and I’ve become very used to the feeling of disappointment that can accompany new experimental techniques. Things rarely work out the first time around, so to see those little spheres straight away was magical.
Dotted across the diamond surface is another, extra, gem. To keep protein nice and happy, you must prepare it in a salty solution. So, when the protein was deposited, some regular table salt, NaCl, came too. We can see this salt in our images as crystals in two distinctive and very beautiful patterns which you can see in the images below.

Protein on the surface of my diamond. Each small pale circle is one of these spherical proteins.
The first image shows a large creeping pattern, reminiscent of snowflakes or tree roots, which spreads its soft fingers across several hundred nanometres. These crystals have taken on an amorphous pattern, where the crystal structure is broken up rather than being one continuous arrangement of the atoms. The second pattern however, shown in the right image, is what a continuous NaCl crystal looks like. When large enough crystals can form without becoming amorphous they look like precise cubes of various sizes all strewn about.
One of my favourite aspects about looking at very small things, is how the patterns you see often mirror those at much larger scales. Look at a fingerprint and you’ll find mountains and valleys, or the roots of a tree and you’ll see a river system.

Salt (NaCl) can take on a highly ordered structure shown by the cubic crystals (left) or an amorphous pattern similar in shape to tree roots (right).
The astonishing images we get from this single session are all in a day’s work for Anders. He has imaged numerous kinds of cells on all manner of interesting substrates, patterned surfaces covered in needle-like protrusions, and many kinds of man-made materials. Today, there are vials on his prep-bench which, at first glance, look much like jars of hair. However, they are not hair, in fact they are strands of carbon fibre covered in various coatings, awaiting examination.
‘What are your favourite types of samples to look at?’ I want to know.
“Cell biology is fascinating,” he says.
“We’ve imaged red blood cells, pancreatic cells, stem cells, and various bacterial cells in this microscope. Most often researchers are interested in cell life and death, and the HIM assists by providing high resolution images of the structure and surface topography of the cell membrane.”
Recently however, Anders has been helping researchers look at polymer materials for water filtration.
“These are hierarchical porous structures, meaning they’re engineered to have pore sizes that vary through the membrane. It is stunning to see the materials at low magnification with large pores, and as we zoom in and in and in, to see new pore sizes become visible at each level, like a material engineered with a fractal quality.”
One of the unique things about the HIM, Anders reminds me, is that it’s not just for imaging. Since helium ions are heavy, they carry a higher momentum than electrons.
“We leverage the momentum of the ions to actually modify structures too. We can create new surface properties, new devices, new technologies, on a scale that is often too small for any other fabrication technique. This is some of the most exciting work.”
If you know anyone who needs some nanoscale drilling done, then the HIM is your instrument!
Today’s excursion across the university campus has been thrilling. I got what I came for and I’m excited to find other projects that could benefit from the insight and beautiful images the HIM can provide. Imaging instruments have always fascinated me and I’m looking forward to witnessing how far we will be able to delve into the nanoscale world in the years to come, thanks to the fast pace of engineering and physics research.